Patterned ground, formed by the arrangement of soil and rocks into periodic patterns from repeated freezing and thawing of ice, has proven difficult to understand due to the geologic timescales involved in their creation. Now, a global team of scientists has investigated this mystery through the lens of phase separation theory using experiments and simulations. Their findings provide new insights about the mechanisms underlying the evolution of these curious and environmentally relevant ground patterns.
In line with human’s tendency to notice and appreciate patterns, it comes as no surprise that patterned ground has captivated the attention of scientists for decades. Coined in 1950, the term “patterned ground” refers to the arrangement of small rocks and soil in symmetrical forms resembling circles, polygons, stripes, and labyrinths. They are typically found in the polar and subpolar regions, including Canada, Alaska, some Soviet Arctic islands, and rocky areas in Antarctica, and have even been observed on the surface of Mars!
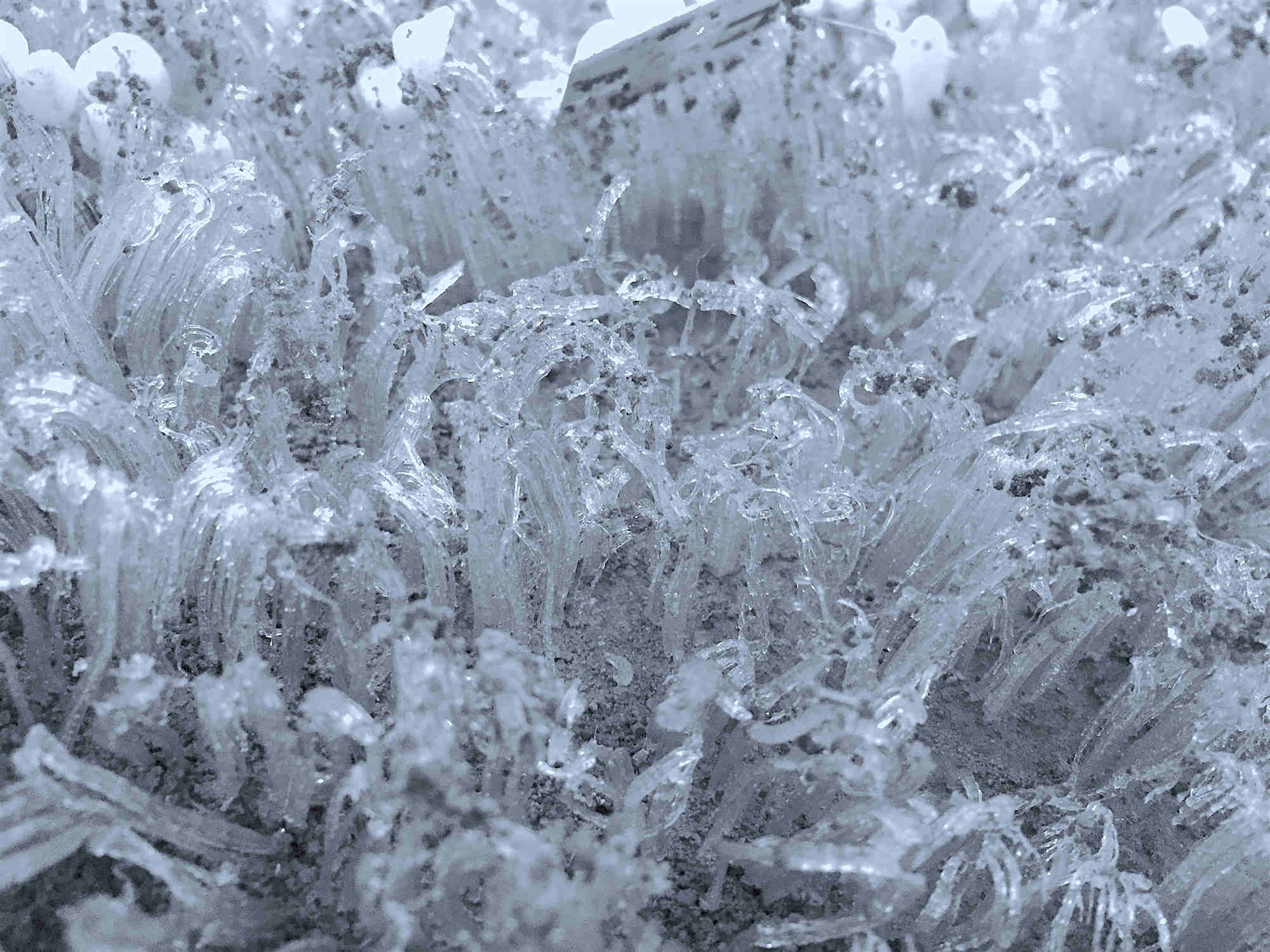
Patterned ground is a remarkable example of self-organization over geological timescales (thousands of years), with repeated freeze–thaw cycles as one of the key ingredients of their formation. As ice forms and melts on the ground, the rocks in the soil tend to aggregate or disperse depending on their size, thanks to the complex interactions between heat transport processes and the surrounding water. However, since the patterns take over thousands of years to evolve, the finer details of the physical mechanism of their formation remain controversial and difficult to investigate.
In a recent study published in
Proceedings of the National Academy of Sciences of the United States of America, a team of scientists led by Professor
Quan-Xing Liu of East China Normal University, China, approached the mystery of patterned ground from a different perspective: phase separation theory. They conducted laboratory experiments as well as numerical simulations to draw insight into how the repeated formation and collapse of ice needles move small rocks in ways that lead to the emergence of the patterns. Then, using two mathematical models, the team showed that the movement of stones depends strongly on their local concentration, and it is this characteristic that drives the formation of patterns in a manner similar to the formation of distinct phases in bimetallic alloys!
The experiments consisted of dispersing small stones over an area of wet soil and subjecting the system to repeated freeze–thaw cycles. The researchers used cameras and software to track the position and velocity of the stones, enabling them to perform detailed statistical analyses. They compared these experimental results with those obtained via numerical simulations using two different mathematical models; one that calculated the motion of stones based on their concentration gradient, and another that additionally accounted for the height of the ice needles formed during the freeze–thaw cycles.
Based on the experimental and simulation results, the phase-separation models put forth by the researchers could successfully represent the formation and evolution of patterned ground. Moreover, depending on the initial conditions, such as stone concentration, freeze–thaw cycle configuration, and ground inclination, they could recreate various types of patterns. “We could reproduce a diversity of patterns typically observed in the field such as Swiss Alps and Haleakela on Maui (Hawaii), including stone circles, labyrinthine patterns, and islands on level ground, as well as stripe-like arrangements on sloped ground.”comments Professor Liu. Furthermore, through a detailed analysis of the results, the scientists gained insight into the dynamics and system feedbacks that give rise to self-similarity and, ultimately, patterned ground.
But why is understanding patterned ground important? For starters, the observation of patterned ground on Mars could be an evidence for the presence of liquid water on the planet at some point in the past. Thus, understanding them could lend new insights into processes that shape the surface of planets over long timescales. Beyond our own planet, patterned ground has been observed on multiple planets in our solar system, from the most inner to the outer (Pluto). But, more importantly, patterned ground can reveal information about the impact of climate change, as Professor Liu explains: “Changes in patterned ground can signal subsurface changes in the vast permafrost regions of the warming Arctic, where instrumentation is extremely sparse at best. Furthermore, visible changes in patterned ground could provide important clues about the release of greenhouse gases from the permafrost to the atmosphere.”
Let us hope a deeper understanding of patterned ground enables us to look beyond its aesthetic beauty and appreciate its significance, both here and on other planets.
Editor's note: the study was supported by STEP, a TPE-related science project.